Collecting Data from Patients with Advanced Diagnostics Tests and Continuous Health Monitoring (The Century of Biology: Part I.IV)
Continuous health monitoring devices embedded in everything from your watch to bed to toilet will track your heart rate, temperature, exercise level, sleep, microbiome, etc. to get a complete picture of what you look like in a healthy state on a day-to-day basis, while also remaining continually vigilant for the first signs of disease. Aggregating these intra-second measurements across continent-sized cohorts of people will allow researchers to understand what it means to be healthy across humanity’s full physiological diversity, follow as people transition from health to illness, predict disease onset before symptoms or contagiousness with increasing accuracy, and help tailor daily habits like nutrition, exercise routine, and sleep patterns to your exact physiology.
Second, when one becomes concerned about an illness, a coming range of highly precise and minimally invasive diagnostics tests will understand what’s wrong, sometimes from the comfort of your home. In particular, for severe diseases like cancer, the advancements in the various -omics described in the sections above has increasingly enabled the study and characterization of such diseases at the inter- and sub-cellular levels. For example, we’re now sequencing the DNA of cancer tumors to characterize it at the level of individual base pair mutations. Aggregating this data on tumor sequences and how they mutate over time will drive our understanding of disease progression and ultimately enable the treatment of said disease based on molecular phenotypes.
The combination of continuous health monitoring and highly specific, minimally invasive diagnostics tests will unleash precision medicine where medical decisions are made based on your physiological measurements, not the average of all of humanity. It will cause the data generated about both health and disease to explode and with it our understanding.
- Diagnostics Tests
Advancements in areas like DNA sequencing, microfluidics, and synthetic biology have enabled less invasive diagnostics tests with vastly improved sensitivity as well as fundamentally new approaches to diagnosing and treating diseases like cancer.
Since cancer is driven by mutations to cells’ DNA, next generation sequencing has been transformational to the field, facilitating early detection and personalized treatment based on the exact progression of the disease. 60-90% of cancer deaths occur because it has spread beyond its original location. Catching cancer early in its progression can improve a patient’s survival odds by 3-14x, as seen below. Dozens of companies[1] are using milliliters of blood samples to screen plasma for circulating tumor DNA. For instance, Personalis’ assay uses 1 mm3 of tumor tissue sampled from a patient to perform whole genome sequencing to identify up to 1,800 single-nucleotide variants. These patient-specific mutations are used to design a panel of primers targeting those regions for sequencing in future blood biopsies. The assay also includes primers for other known cancer-related genes. Meanwhile, academic researchers are figuring out how to apply AI to sequencing data to get the most signal out of the smallest samples. One recent paper improved sensitivity to parts per million range. Additionally, some companies combine genomic data with proteomics, immunprofiling, etc. for a multiomic approach. That will likely increasingly become the standard of care.
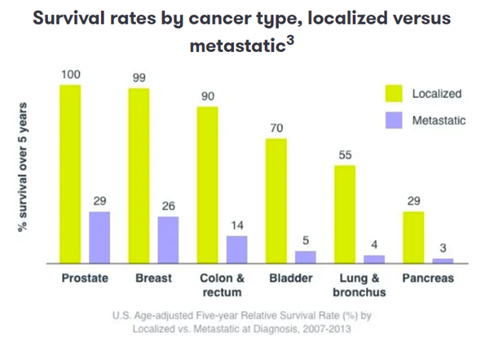
Our approach to treatment can be improved not just by addressing the disease right away but also by tailoring treatment to individual patient’s situation and the genetic specifics of their tumors. As these companies analyze ever larger scales of DNA sequences from each patient’s tumor as it grows and as it reacts to a given treatment, they’ll gain expertise in treating a given patient with a given genetic mutation. Indeed, companies like Personalis are already doing this, using their whole genome sequencing and screens for known cancer-related genes to “see if there are other variants emerging that have a therapy that may be more effective for that patient.”1
Here are some other diagnostics advancements being made outside of cancer:
Their diagnosis thus far has mainly relied on surgical biopsies to obtain tissue that is then analyzed – an invasive process that also often disrupts and degrades the chemistry of the samples. To overcome these issues, Core Faculty member Samir Mitragrotri, Ph.D., and his team at the Wyss Institute are developing non-invasive sampling techniques that can quantify diagnostic biomarkers from patients’ skin without the need for a biopsy. The obtained samples are suitable, for example, for detecting UV-induced damage to the DNA of skin cells to assess the risk of skin cancer, and analyzing changes in skin chemistry that can indicate internal disease.
Wyss Institute has developed freeze-dried synthetic gene networks that can detect disease-specific nucleic acids, and then printed them onto paper along with freeze-dried gene expression machinery that can be activated by just adding water. Their approach successfully identified molecules of the Zika and Ebola viruses as well as pathogenic bacteria with high sensitivity and specificity.
Wyss is also developing sensitive approaches to isolate exosomes – small, membrane-enveloped particles secreted by many cells of the body, including the brain – for use in non-invasive diagnosis of neurological and cognitive disorders, such as Alzheimer’s and Parkinson’s disease. Exosomes continuously circulate in the blood and contain molecules that may signal diseases present in the cells and areas from which they are released, offering the potential for early, less invasive diagnostic tests of brain and other diseases, as well as the possibility of therapeutics that, when given earlier in life, could suppress neurodegeneration in older age.
It’s also pioneering techniques that can measure extremely low concentrations of already accepted or potential biomarkers from patient samples down to the single-molecule level. To do this, they designed a competition strategy in which a patient-derived biomarker molecule is tagged and competes with an unlabeled version of the same molecule in the patient sample to bind to antibody molecules attached to plastic microbeads. The tagged molecules are then identified by an enzymatic amplification strategy performed in microwells. The ratio between fluorescent and non-fluorescent microwells is then used to calculate the concentration of the biomarker with 50-fold greater sensitivity than conventional detection methods. As this technique can sense the concentrations of many types of molecules whose levels change over the course of disease, it is a versatile, highly sensitive diagnostic instrument that could improve diagnosis of many diseases. Walt’s team is working now with Wyss Core Faculty member David Weitz, Ph.D., to convert the present single-molecule assay into a drop-based microfluidic format to enable point-of-care applications.
Expanding the use of portable PCR testing: 3EO Health is developing an at-home molecular testing with PCR-grade sensitivity and antigen test-level cost. Visby provides rapid PCR tests for STDs and respiratory diseases with results in <30min. This changes the paradigm for STD testing from an embarrassing, tedious process of going into the doctor’s office to one as easy as COVID testing.
Biosensors that can detect singular molecules, improving for example early detection of cancer:1 “The MoS2 biosensors demonstrated by the UCSB team have already provided ultrasensitive and specific protein sensing with a sensitivity of 196 even at 100 femtomolar (a billionth of a millionth of a mole) concentrations. This protein concentration is similar to one drop of milk dissolved in a hundred tons of water. An MoS2-based pH sensor achieving sensitivity as high as 713 for a pH change by one unit along with efficient operation over a wide pH range (3-9) is also demonstrated in the same work. "This transformative technology enables highly specific, low-power, high-throughput physiological sensing that can be multiplexed to detect a number of significant, disease-specific factors in real time," commented Scott Hammond, executive director of UCSB's Translational Medicine Research Laboratories. "This invention has established the foundation for a new generation of ultrasensitive and low-cost biosensors that can eventually allow single-molecule detection—the holy grail of diagnostics and bioengineering research.”
- Devices for Continuous Health Monitoring
Seven decades of Moore’s Law has brought us supercomputers on people’s wrists with a half dozen different clinical-grade health trackers. Beyond smart watches, people are increasingly thinking of things they physically interact with every day from their bed to their toilet as not just innate objects but ones capable of collecting treasure troves of data on their health, in the background, without them having to think about it. We now have beds that track sleep and can automatically adjust mattress temperature in response; toilets that scan for signs of illness, nutritional deficits, microbiome analysis, and cancer screening; patches to continually track blood glucose; smart tampons tracking reproductive health; keyboards that scan for Alzheimer’s; among many others. Indeed, patents for biomarker devices have risen exponentially over the last decade, hundreds of companies have been launched, and hundreds of millions of people are now using them. The advent of continuous health monitoring, as embodied by devices that collect over 250,000 data points a day1 on continent-sized cohorts, enables a fundamentally new approach to studying and promoting health. We can study what healthy looks like instead of only those that in need of immediate assistance; we can establish individuals’ baseline health such that deviations from it would flag rising health risks; and, best of all, we can track the progression of disease from start to finish. Again, all this can happen with unprecedented granularity and scale.
The most famous of all continuous health devices, the smart watch, keeps gaining new health-tracking functionality and has been validated by independent researchers to produce clinical-grade data. The Apple watch’s first health feature, heart rate tracking, has been shown to be reliable for daily activity1,2 and even more extreme conditions like intense exercise1 and atrial fibrillation monitoring1. It tracks background heart rate using infrared light and high intensity work outs via LED lights flashing hundreds of times a second. More recent models take heart monitoring a step further using electrodes to read the heart’s electrical signals (ECG/EKG). These electrodes are built into the Digital Crown and the ring on the back of the Apple Watch such that “when you place your finger on the Digital Crown, it creates a closed circuit between your heart and both arms, capturing the electrical impulses across your chest,” Apple explains on its support page.
All told, over 1,000 clinical trials for consumer remote heart monitoring have been started, 13 devices have been approved by the FDA, and half of the 67 million US smart watch owners intend to use them track their cardiac health.1 Heart rate monitoring and skin temperature sensors have been shown to be useful for predicting a wide variety of disease states:
- Cardiac issues: A large number of studies have been performed recently to assess the ability of machine learning algorithms to analyze HRV in specific cardiac applications. The accuracy was modest to excellent, based on the application: arrhythmia and atrial fibrillation detection (10,000+ studied patients, DT or CNN, 95–100% accuracy)63,64,65, long term cardiovascular risk prediction (859 patients, eXtreme Gradient Boosting, 75.3% accuracy)66, sudden cardiac risk/ventricular fibrillation prediction (100+ patients, SVM or ANN, 67–89%)67,68,69, hypertension detection (209 patients, RF had the best accuracy of 86%)69. The accuracy of HRV-based risk stratification for each indication is affected by the amount the vegetative nervous system contribution to the pathophysiology of these conditions. (Source)
- Diabetes: One of the dreaded complications of diabetes mellitus is autonomic neuropathy, with resulting increase in cardiovascular morbidity and mortality. As the autonomous nervous system may be affected early in the disease course, HRV analysis can be used to detect diabetes or monitor the efficacy of treatment. Diabetes detection had good accuracy in small studies (200+ patients, CART or GPC, 84–93%) [76,77]. Changes in HRV detected hypoglycemia in a study of 23 patients using a wearable device (VitalConnect HealthPatch) with 55% accuracy [78].
- Illness:
By using wearable devices to measure things such as heart rate and skin temperature, which are known to elevate when the body is fighting off an infection, the team seeks to train a series of algorithms that indicates when your immune system is acting up. When people come down with a cold or flu, there’s usually a period just before symptoms set in when they wonder if they’re actually getting sick. Even during that time, without heavy symptoms, a sick individual often can still spread the virus. “You might wonder, ‘Are these sniffles allergies, or am I getting sick?’ These algorithms could help people determine if they should stay home in case their body is fighting off an infection,” Snyder said.
Snyder also foresees some kinks to work out. “It’s possible that the algorithms could detect an elevated heart rate, but the user could be watching a scary movie or participating in some other activity that naturally elevates heart rate,” he said. “An alert isn’t a direct diagnosis, and it will be important for folks to be able to contextualize their situation and use some common sense.” Snyder also adds that even as his team works to develop algorithms that can flag illness, the next step is to investigate whether those signals can be sorted to be able to differentiate between viruses. (Source)
- Baseline health:
The idea of this study was to monitor ordinary, relatively healthy people; get a good idea of their biological norms, such as their heart rate, blood pressure, immune molecules and gene expression; and then watch for changes that might hint at a shift in health. Of the 109 participants, the majority of the group had a clinically actionable outcome. In other words, some readout, be it from a blood test or smartwatch, flagged a potential health problem that was treatable or manageable. None of these issues had been detected before, Snyder said.
“We caught a lot of health issues because we noticed their delta, or their change from baseline,” he said. “For instance, we caught nine people with diabetes as it was developing by continuously monitoring their glucose and insulin levels.”
Through genetic sequencing, the team identified 13 disease-related findings, two of which were associated with serious heart defects. “The person was quite young, and we found that they had a mutation in a gene that puts you at risk for cardiomyopathy, which causes problems for the heart muscle,” Snyder said. “And sure enough, subsequent cardiac testing proved this person had heart disease.” The list goes on: 18 people discovered they had high blood pressure; two people had precancers. Multiple people found out they had low hemoglobin, and six participants had arterial plaques. (Source)
- Predicting the results of more invasive clinical procedures:
A smartwatch can signal physiological changes, such as a change in red blood cell count, as well as early signs of dehydration, anemia and illness, according to a new study led by researchers at Stanford Medicine. The study is among the first to show that smartwatch data correlates with laboratory test results.
“We wanted to know if smartwatch-derived signals could predict the same values without any invasive intervention.” The answer was a resounding yes. Certain combinations of smartwatch-derived data were able to provide insights into specific clinical blood tests. Changes in heart rate, for instance, often predicted changes in red blood cell count and hemoglobin, the molecule that carries oxygen. Researchers also saw that participants with a rise in skin temperature and a decrease in movement generally also had a higher immune cell count — an indication of an illness such as a cold or the flu. (Source)
In addition to heart rate monitoring, smart watches have been shown to be accurate sleep trackers, some comparable to in-lab polysomnography, the gold standard in the field.1 Deep learning-based methods can be highly accurate in sleep apnea detection (10,000+ patients, ANN or CNN, 85–89% accuracy)74,75.
Beyond the already launched monitors tracking background heart rate, ECG / EKG, HRV, sleep, step count, calorie expenditure, temperature, and blood oxygen, Apple has had plans dating back to the Steve Jobs era for its watches to feature non-invasive continuous glucose monitoring that relies on absorption spectroscopy or photonics so as to not require a blood draw. It also has patents for blood pressure monitoring and the next year’s version is rumored to boast such tech.
As Moore’s Law marches on and as sensory systems continue to improve, these smart watches will only continue to get more accurate and incorporate more monitoring features. And yet, smart watches are only the tip of the iceberg when it comes to continual background health monitoring. Tens of billions of dollars have been deployed from the top VC firms and from the major multinationals in tech (e.g. Apple, Samsung, and Google), appliances (e.g. Samsung), and healthcare (e.g. Abbott, Dexcom) into ventures that embed health measurements into all the goods we interact with on a daily basis.
Several companies have launched smart beds that track your sleep based on your whole body’s movement throughout the night, that identifies optimal mattresses temperature for you and can automatically adjust it if you happen to get feverish sweats one night. Within sleep tracking, one could also imagine augmented eye masks that use computer vision to track eye movement to better capture sleep stages or maybe even ones that use technology similar to this company’s forehead patch to track brain waves or apply in-ear EEG tracking technology to ear plugs like this company. Users could integrate data from their Oura ring or their smart watch with their bed / eye mask / ear plugs to get highly specific sleep tracking data.
One of the most exciting (and goofy) health monitoring devices are smart toilets. Number one and number two are treasure troves of health data, maybe even more valuable to health diagnostics than what can be measured by a smart watch. Researchers from Stanford to Duke and half a dozen startups[2] are taking porcelain bowl technology beyond bidets to mine stool and urine for relevant data. Their products use physical and molecular tests to determine urine flow rate, stream time, total volume, number of visits to the loo a day, stool consistencies and color, white blood cell count, consistent blood contamination, various protein level, etc. This can screen for a broad spectrum of diseases from infectious diseases like COVID to bladder and colon cancer to kidney failure, improve diet analysis, and help patients with irritable bowel syndrome or Crone’s disease manage their diseases by for instance monitoring flare ups in real time and recommending adjustments to medicine doses to the patients’ physician. The Duke team is combining that physical and molecular analysis with biochemical one to be able to analyze your microbiome, though such a solution may be pricey1.
Another major category with already mature offerings is continuous glucose monitoring (CGM). Major healthcare companies and a dozen well-funded startups have launched GCM patches in the last couple years[3] to treat diabetes as well as for healthy people to optimize their nutrition. Consider the progress this category has made1. Formerly, to get such a health reading, you’d have to go into a clinic, have 20mls of blood drawn. Now you can put a patch on your arm with a miniature needle that continuously measures your blood glucose using tiny amounts of blood. And, eventually, Apple will release an updated watch model that doesn’t require any blood drawn or a separately worn patch.
Here are other examples:
- Abbott’s developing patches to track ketones continuously to measure when users are in the fat burning state called ketosis to eliminate confusion on if a diet / weight loss program is working. They’re also making a device to continuously track lactate build up during exercise, as an indicator of athletic performance.
- A group at CalTech devised a ring-like wearable to continuously track female hormone levels continuously. Nanobiosensors analyze aptamers in sweat to automatically monitor estradiol.
- NextGen Jane uses a patented smart tampon technology to collect menstrual and cervicovaginal samples used to conduct diagnostic tests for reproductive healthcare conditions.
- Stanford’s Precision Health and Integrated Diagnostics Center is working on the following projects:
- A smart bra that constantly images breast tissue through photoacoustic principles to detect the earliest possible signs of breast cancer. This is also being pursued by MIT.
- A magnetic needle that safely resides within your bloodstream to sample the potential presence of rare molecules being shed from diseased cells.
- New microscopes can image single cells in your body without removing tissue from your body.
- New clinical longitudinal studies to sample molecules from humans over long periods of time in order to define the meaning of human health.
- Strategies to sample small molecules in your breath looking for the earliest signs of disease.
- Muscle contraction trackers for optimizing work outs
- A group that used ML to predict the early stages of cognitive diseases like Alzheimer’s based on typing patterns on computer keyboards or smartphone touchscreens. “We measure that precise moment, in milliseconds, when your brain and finger interact with the device,” explains Bavasso. “Let’s say your finger is on the ‘L’ key, and your brain is sending a signal to move the finger to the ‘P’ key. Patients who have Parkinson’s will experience a delay in this signal, leading to a lag in the keystrokes.” ML algorithms can identify such patterns from many types of device interactions, such as how hard a person presses keys, their reaction time to images on a touchscreen, and how often they use the backspace. “Since we use our devices eight to ten hours a day, a brain health score iterated every 90 seconds gets refined over weeks or months, leading to pretty high accuracy,” adds Bavasso. The group’s approach using devices we interact with every day delivered a 33% improvement in diagnostic accuracy over the current gold standard test1 and has been FDA approved.1
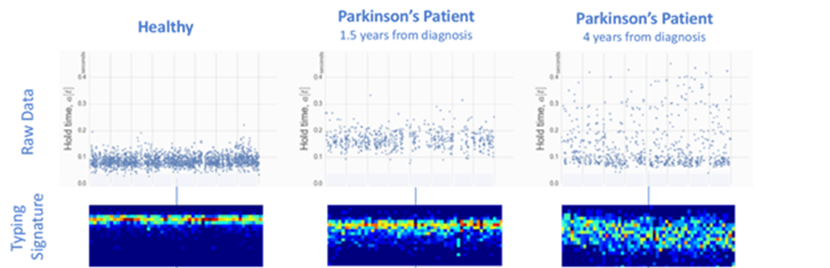
Once again, these devices have hundreds of millions of users, are fast growing markets, and several products are already seeing commercial success, with Apple watch generating $8B in revenue last year, Abbott expecting its CGM to become a $10B product in five years1. Funding for the technological advancement of these products and consumer comfortability with them will only rise.
Looking at these devices and the trends behind them, one can trace the ceaseless march towards these products getting embedded deeper into the background of our lives. This trend follows that of consumer tech at large: it went from main frames to desktops to PCs to iPhones to watches to AirPods. Biosensors are a couple decades younger in terms of its technological maturity, but the fun part is they’re starting to blossom on their own and exit their awkward tween years. The end game for them is to be embedded into the background so seamlessly that you don’t even notice or think of them as they continuously make sure you’re as healthy as can be. A perfect encapsulation of that vision is a concept laid out by the CEO of Oxford Nanopore for a miniaturized DNA sequencer inside your toothbrush, scanning every day for cancer, microbiome imbalances, etc:
An ideal device would be a toothbrush, I think. There's no reason why we can't have a small integrated sequencer that would fit inside a toothbrush. And when you clean your teeth, if you clean them well, there should be a little bit of blood. You're basically opening up cells and releasing the DNA. In the mouth you have 50,000 white blood cells per mL. When you scrape your teeth, you release around 100mL of serum or blood. Doing that twice a day if you sum over the stuff you are measuring, you're looking at 10, 20mL of blood, in tiny steps. We can select molecules, filter molecules, if we see a molecule that looks suspicious, we can sequence it again and again and be certain that we're seeing a pathogenic mutation. That all sounds completely bonkers but that's where I want to take this technology over the next 20, 30 years.
Such a device is a twice-a-day liquid biopsy, continually vigilant for signatures of cancerous free-floating DNA. This would extend the revolution currently taking place in DNA-based cancer detection and treatment, changing the paradigm from in person doctors’ visits to such testing happening passively in the background. It would go a long way towards making late-stage detection of cancer a thing of the past. It could also team up with your smart toilet to analyze your microbiome by sequence the bugs in your mouth. Lastly, he makes it clear that it’s a generalizable approach because “when your immune system starts to react to something, your RNA expression levels change. So, it could be something very simple, that you can see just like temperature, or blood pressure shifting, it's very generic, but it's very broad.”
Continuous health monitoring and diagnostics may even continue its march towards miniaturization all the way into the body. We already have examples of implantables for at risk patients, like pacemakers the size of pills that control the rhythm of the heart and transmit ECG data to the patient’s smartphone or sensors in replacement parts like this ‘smart knee implant’ that helps patients adjust their gait. Whether or not implantables become palatable to people without an immediate health need, they demonstrate that monitoring from withinside the body isn’t out of the question. And, as work in nanotechnology has heated up over the past two decades[4], we’ve started to see glimpses of what non-invasive monitoring from within might look like.
- Pamela Silver, Ph.D., also one of the Wyss Institute’s Core Faculty members, and her team in the Institute’s Living Cellular Devices platform are engineering bacteria to function as disease indicators when added to the gut microbiome. These “Living Cellular Diagnostics” are engineered so that they can sense, for example, inflammation in mice similar to inflammatory bowel or Crohn’s disease and produce a signal molecule that can be analyzed non-invasively through fecal samples.
- Micro robots that can measure aspects of cells1,2. For instance, this one converts magnetic field signals into sound waves to be able to safely communicate from withinside a cell:
Recent engineering advances have enabled scientists to shrink electronics down to the cellular scale—with hopes of potentially using them to explore and manipulate the innards of individual cells. But such a rover would need to receive instructions and transmit information—and communicating with devices this small can be extremely difficult. “Miniaturizing an antenna to fit inside the cell is a key challenge,” Sarkar says. The problem involves the electromagnetic waves that are used with most conventional antennas, like those in cell phones, to transmit and receive data. Antennas operate best at their so-called “resonant frequencies,” which occur at wavelengths roughly equal to the antenna’s actual length. Because of the mathematical relationship between a wave’s speed, frequency and wavelength, waves with shorter wavelengths have higher frequencies. Unfortunately, subcellular antennas have to be so tiny that they require frequencies in the microwave range. And like the beams in a kitchen microwave, these signals “just fry up the cells,” Sarkar says. But she and her colleagues think they have a solution. In a Nature Communications paper, they describe a new antenna design that can operate safely inside cells by resonating with acoustic rather than electromagnetic waves. A functioning antenna could help scientists power, and communicate with, tiny roving sensors within the cell, helping them better understand these building blocks and perhaps leading to new medical treatments.
While inside the cell, the Cell Rover was able to receive an electromagnetic transmission and send a responding signal outward, up to a distance of one centimeter. The researchers also added multiple different-sized Cell Rovers to a single cell, and found they could distinguish the transmission signals of individual rovers.
So far the scientists have only showed that the Cell Rover can work in principle, using it to send empty signals; this type of transmission can be thought of as being a little like static on a TV. Next they will try to determine what kind of “shows” they can watch by outfitting the rover with tiny instruments that could collect and convey information about the rover’s surroundings. For instance, they might add a simple polymer coating that would bind to nearby ions or proteins. When these substances stick to the polymer they would change the Cell Rover’s mass, and this in turn would alter the acoustic vibrations it produces. By measuring these changes, researchers could assess a cell’s protein or ion levels.
“The Cell Rover is an innovative concept as it can embed sensing, communication and information technology inside a living cell,” says Anantha P. Chandrakasan, dean of the MIT School of Engineering (Source)
Whether or not such sci-fi technology becomes commonplace, one thing’s for certain: we’re going to see biological sensors get ever-more multimodal, more passively embedded into the background of our lives, and more accurate and precise.
Conclusion
The trends described above will bring about not just a revolution in health but in healthcare and its associated costs. Currently, you go into the doctor’s office only after you feel ill enough to make the trek to the office generally at some inconvenient time and wait for the seven people in front of you to be seen. That costs you hours out of your day and costs the healthcare system hundreds of dollars’ worth of a highly trained professional’s time. Moreover, the actual medical detective work hasn’t changed much over the last half a century. It may include taking your temperature and blood pressure at that particular moment, tapping a rock against your knee, asking some questions about how you’re subjectively feeling, or sticking a needle in your arm to draw a quart of blood. We don’t make use of currently available technologies like DNA-based screens for genetic diseases or predispositions.
From the physiological measurements collected, the doctor then makes decisions about your health based on comparing it to population averages. Here, the adage that you shouldn’t cross a river that’s on average 5 ft deep applies because everything about people’s body is on a continuum, not just height and weight. For instance, we’re told that our proper temperature is 98.6°F, but that’s a (possibly erroneous) estimation of the human average. In reality, temperature varies widely by person. A study of almost 3,000 people found that it ranges from 92.0°F to 100.8°F with a 25th percentile of 94.6°F and a 75th percentile of 99.1°F.1 So, let’s say your personal average temperature is 94.6°F, if you go into the doctor’s office and measure 98.6°F, they’ll tell you you’re perfectly healthy, when in reality you could be having a fever.
Continuous health measurement will transform every aspect of that picture. Health monitoring will happen in the background, continuously. You’ll know your own personal averages for all physiological datapoints, track when something’s out of whack. This alert may happen well before you’re symptomatic – think of Michael Snyder’s group using an Apple watch alone to predict COVID a median of 3 days before symptom onset.1 And, the illness or issue may be diagnosable remotely based on your device data or with the types of highly accurate at home diagnostics tests described above.
We’re transitioning from this fundamentally broken model that’s like putting out a fire once it’s starting to burn the whole house down to one that uses the most sophisticated micro technologies to continually scan for threats and puts out the fire immediately. We’re transitioning from sick care to health care.
Read next section: organ-on-a-chip to simulate biology at scale
[1] Harbinger Health, Guardant Health, Personalis, Grail, ArcherDX (acquired for $2.3B), Haystack Oncology (acquired for $450M), Freenome among others
[2] These include Kanaria (launched by Stanford’s research team), Coprata (spun out of Duke), Toi Labs, OutSense, Olive Diagnostics (raised $15M), Yellosis (backed by Samsung)
[3] Healthcare multinationals include Abbott and Doxcom. Startups include EOFlow (acquired for $750M), Levels (raised $100M), Intuity, PHC Group, Beta Bionics, Sibionics
[4] See here for a summary of work to make miniature battery-free bioelectronics.